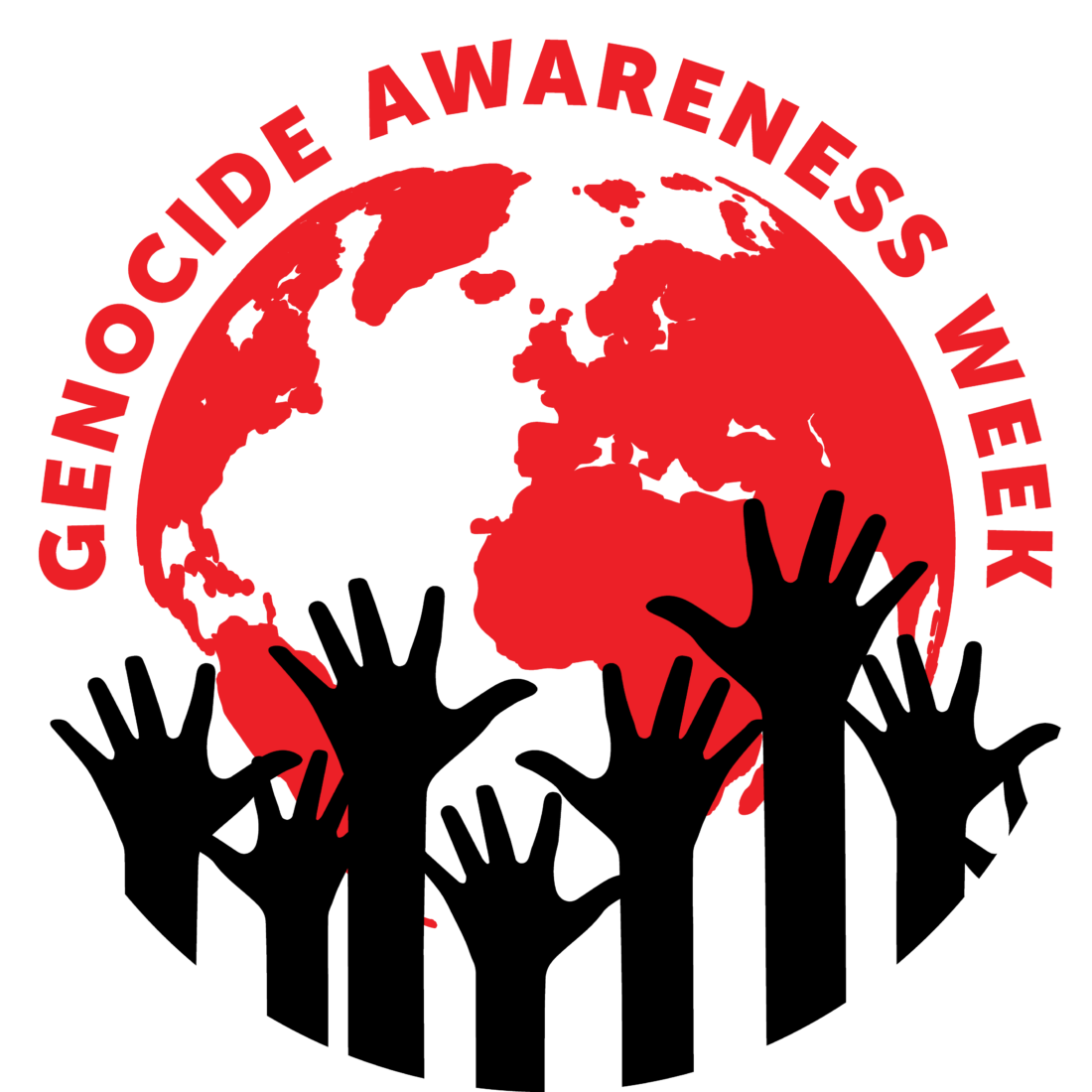
(Source: ASU)
Last week’s blog was focused on learning, from the Warsaw Ghetto under Nazi occupation, how to try to oppose a deadly hostile environment in a non-violent manner. The proposed remedy was to create a modern (computer-based) Ringelblum archive that is opaque to the hostile environment, and that can be opened when the political environment gets more friendly. At the end of that blog, I promised to show an example in this blog. Not surprisingly, the examples that I will use relate to the current global trends that may lead to existential global threats. Collectively, I define the processes as “self-inflicted genocide.”
Before I go into these trends, I need to discuss two important elements in the concept: my degree of certainty in its continuation and the embedded assumption of “business as usual,” without which we might mitigate the crisis. The best entry to these uncertainties is through an invited talk that I gave on December 1, 2016, at the University of Pennsylvania. The assembly aimed to convince the University to disconnect from investing in fossil fuels. I was invited by a student, Richard Ling, who—together with Thomas Lee—wrote a detailed paper summarizing the concept. I strongly suggest that you read the full manuscript. Here, I am including part of their summary that includes my position on the issue:
As a Holocaust survivor and professor of physics at Brooklyn College, Micha Tomkiewicz has a nuanced understanding of genocide in the context of climate change. In a talk at the University of Pennsylvania on December 1, 2016, Tomkiewicz painted climate change as a prospective “self-inflicted genocide.” With greenhouse emissions (whose increase is primarily attributed to fossil fuel combustion) threatening irreversible harms to ecosystems, living organisms, and the human race, Tomkiewicz makes it salient that his comparison of climate change to genocide should be used as a marker of direction and pointer to clear evil. He warned: “It’s easy today to teach students to condemn the Holocaust, but it’s much more difficult to teach them how to try to prevent future genocides.” As moral agents, we ought to maintain an active memory of past injustices and proactively act in the face of new moral evils. Truth and justice ought not to be dismissed in 19 words.
Following the talk, I wrote a blog that summarizes my position on that event, including some relevant information not included by the students’ summary:
Richard’s request forced me to take another look at my claim that by the end of this century the impact of climate change would amount to “self-inflicted genocide.” I decided to see whether such an association didn’t need some narrowing. Reading Philippe Sands’ new book, East West Street: On the Origins of Genocide and Crimes Against Humanity helped me with this reassessment. The word “genocide” was coined by Raphael Lemkin and used in the 3rd indictment of the Nuremberg Trials. The definition used in the trial was: “Extermination of racial and religious groups, against the civilian populations of certain occupied territories in order to destroy particular races and classes of people and national, racial, or religious groups, particular Jews, Poles, Gypsies and others.” From Sands’ book I also learned that Lemkin’s best friend in Poland was my great uncle. I suddenly started to feel even more personal pressure to specify my use of the term in a context that I am almost sure Lemkin would not have agreed with.
The definition of genocide is now moving well beyond Lemkin and the UN’s original definition.
I went to AI (through Google) for a recent summary of the definition:
Genocide is an internationally recognized crime where acts are committed with the intent to destroy, in whole or in part, a national, ethnic, racial, or religious group.
-
- Key Elements:
- Intent to Destroy:The most crucial element is the intent of the perpetrators to destroy the group, not just to harm or persecute individuals.
- Targeted Group:The victims are targeted because of their membership in a specific group, not because of their individual actions or characteristics.
- Acts of Destruction:These acts can include killing members of the group, causing them serious bodily or mental harm, deliberately inflicting conditions of life calculated to bring about physical destruction, imposing measures intended to prevent births within the group, and forcibly transferring children of the group to another group.
- Historical Context:
The term “genocide” was coined in 1943 by Raphael Lemkin, a Jewish-Polish lawyer, who combined the Greek word “genos” (race or tribe) with the Latin word “cide” (to kill).
-
- Distinction from Other Atrocities:
While genocide is a specific crime with a unique intent requirement, it’s important to distinguish it from other mass atrocities like crimes against humanity and war crimes, which may not involve the intent to destroy a group.
The global trends that we are currently experiencing were summarized in a previous blog titled “What Are We Trying to Teach Our Children?” (June 11, 2024). A key paragraph from that blog is given below:
Humanity is in the middle of at least 5 existential transitions; all of these started around WWII. They include climate change, nuclear energy, declining fertility, global electrification, and digitization. These transitions started around the time that I was born, but they will hopefully last (if some of them do not lead to extinction in the meantime) at least through the lifetime of my grandchildren (I call this time “now” in some of my writing).
For the purpose of this blog, I will now change the word “hopefully” to probably.
In “business as usual” scenarios, some of these trends could lead to global genocide. Such trends include: climate change, global epidemics, nuclear war, a large asteroid hitting Earth, and the digitization that has led to AI.
All of these threats are projected to take place in the future. Some of these threats are the same age as Lemkin and myself (digitization/AI, climate change, and nuclear war) while two others have a longer history (global epidemics and a large asteroid hitting Earth).
I will go now through the projections of the magnitude of these threats, starting with the newest and “simplest” threat – AI.
AI
About two weeks ago, an article came out describing how AI can revolutionize science:
Across the spectrum of uses for artificial intelligence, one stands out.
The big, inspiring A.I. opportunity on the horizon, experts agree, lies in accelerating and transforming scientific discovery and development. Fed by vast troves of scientific data, A.I. promises to generate new drugs to combat disease, new agriculture to feed the world’s population and new materials to unlock green energy — all in a tiny fraction of the time of traditional research.
Technology companies like Microsoft and Google are making A.I. tools for science and collaborating with partners in fields like drug discovery. And the Nobel Prize in Chemistry last year went to scientists using A.I. to predict and create proteins.
This month, Lila Sciences went public with its own ambitions to revolutionize science through A.I. The start-up, which is based in Cambridge, Mass., had worked in secret for two years “to build scientific superintelligence to solve humankind’s greatest challenges.”
Relying on an experienced team of scientists and $200 million in initial funding, Lila has been developing an A.I. program trained on published and experimental data, as well as the scientific process and reasoning. The start-up then lets that A.I. software run experiments in automated, physical labs with a few scientists to assist.
The description of the global threats posed by this technology is much shorter:
I can ask it a “simple” question: “how to destroy the world” or “how to prevent the destruction of the world?” For certain individuals within our 8 billion people, the two opposing perspectives of the question are basically the same. If these individuals have the right prerequisites to be able to put the AI suggestions to work – would the result be considered genocide?
Nuclear War
Threats of nuclear war (often labeled WWIII) are now spreading. The Russians are reacting negatively to almost any step associated with the West’s help for Ukraine. Trump is threatening Zelensky that there will be major consequences if the US continues to defend Ukraine. Meanwhile, the West is trying to strategize on a new nuclear umbrella to replace the US.
The results, if the current nuclear arsenal of both the US and Russia were to be triggered, have been summarized by Bulletin of Atomic Scientists:
A global all-out nuclear war between the United States and Russia with over four thousand 100-kiloton nuclear warheads would lead, at minimum, to 360 million quick deaths.* That’s about 30 million people more than the entire US population.
360,000,000
* This estimate is based on a scenario of an all-out nuclear war between Russia and the United States involving 4,400 100-kiloton weapons under the 2002 Strategic Offensive Reductions Treaty (SORT) limits, where each country can deploy up to 2,200 strategic warheads. The 2010 New START Treaty further limits the US- and Russian-deployed long-range nuclear forces down to 1,550 warheads. But as the average yield of today’s strategic nuclear forces of Russia and the United States far exceeds 100 kilotons, a full nuclear exchange between the two countries involving around 3,000 weapons likely
An asteroid hitting Earth
From Center for NEO Studies (CNEOS):
CNEOS analysis of near-Earth asteroid 2024 YR4, which is estimated to be about 40 to 90 meters wide, indicates it has a more than 1% chance of impacting Earth on Dec. 22, 2032 — which also means there is almost a 99% chance this asteroid will not impact. These analyses will change from day to day as more observations are gathered. The CNEOS analyses are used for NASA’s contribution to the International Asteroid Warning Network (IAWN). After the impact probability for this asteroid reached 1%, IAWN issued its official notification for the potential impact.
Cosmological collisions are not unusual. However, the last one that hit Earth was about 66 million years ago, well before the emergence of humans:
The Cretaceous mass extinction event occurred 66 million years ago, killing 78% of all species, including the remaining non-avian dinosaurs. This was most likely caused by an asteroid hitting the Earth in what is now Mexico, potentially compounded by ongoing flood volcanism in what is now India.
A 1% probability within 7 years leaves a 99% probability of a non-event. But in case that 1% were to grow, a human response would take time. As small of a probability as it is, we can’t discount the effects of the last asteroid.
Epidemics
Covid-19 – 14.9 million excess deaths associated with the COVID-19 pandemic in 2020 and 2021 (According to the UN)
Spanish Flu – (Wikipedia)
The 1918–1920 flu pandemic, also known as the Great Influenza epidemic or by the common misnomer Spanish flu, was an exceptionally deadly global influenza pandemic caused by the H1N1 subtype of the influenza A virus. The earliest documented case was March 1918 in Kansas, United States, with further cases recorded in France, Germany and the United Kingdom in April. Two years later, nearly a third of the global population, or an estimated 500 million people, had been infected. Estimates of deaths range from 17 million to 50 million,[6][7] and possibly as high as 100 million,[8] making it one of the deadliest pandemics in history.
Climate change – New Health Data Shows Unabated Climate Change Will Cause 3.4 Million Deaths Per Year by Century End – V20: The Vulnerable Twenty Group
Unabated climate change will cause 3.4 million deaths per year by the end of the Century, new data presented to COP27 today shows. Health-related deaths of the over-65s will increase by 1,540%, and in India alone there will be 1 million additional heat-related deaths by 2090, if no action to limit warming is taken, the data shows.
Back to the definition and inclusion of the modern Ringelblum Archive. The point is to protect the ideas and arguments that could currently be dangerous to publish in this hostile environment. Inclusions into the archive should include arguments for and against a contribution and it should be used, as all research should be used, as an argument to encourage further research on the issue. In this case, we are talking about the lifespan of infrastructure (e.g. the built environment) that is estimated based on the global threats (e.g. climate change) that the hostile environment (e.g. the government) discounts. In many cases, two versions of the same related issue could be collected; one might be acceptable and publishable in the present environment and the other, with the “speculation” of future consequences, could be stored in the archive.
As I am finishing writing this blog, President Trump is issuing another executive order:
March 19 (Reuters) – President Donald Trump issued an executive order on Wednesday related to state and local infrastructure preparedness for responding to natural disasters such as wildfires and hurricanes, along with other threats like cyber attacks.
“This order empowers state, local, and individual preparedness and injects common sense into infrastructure prioritization and strategic investments through risk-informed decisions,” the order said.
The only way to comply is to make a new, modern Ringelblum Archive that can stay transparent to any administration.
Stay tuned!